Chapter Contents:
- Climate is a System
- Measuring Climate
- Greenhouse Gases and Global Temperature
- Natural Causes of Climate Change
- Summary and Additional Resources
Page Topics: Overview, How Systems Work, Earth's Climate System (Atmosphere, Hydrosphere, Geosphere, Biosphere), and Regional Weather and Climate Systems.
Image above: The four components of Earth's climate system: the atmosphere, geosphere, biosphere, and hydrosphere. Image created by Jonathan R. Hendricks for PRI's Earth@Home project (CC BY-NC-SA 4.0 license).
Overview
One can describe climate as a symphony of weather, taking into account the entire range of weather conditions in a region, the extremes as well as the average.* Climatologists technically define climates through the calculation of “climate normals”: thirty-year averages of variables such as daily temperature, rainfall, snowfall, and frost and freeze dates that can be compared with thirty-year averages of these variables from other time periods (learn more about climate normals here). Fluctuations in these variables that last hours, days, or up to two weeks are called weather.
Weather is what we all feel when we step outside: if you want to know what today’s weather is, just go outside! No one ever actually experiences the climate in the present, because no one can ever step outside and feel that day’s climate. One practical way to think about the difference between climate and weather is that knowing the weather tells you what clothing to wear on a given day, whereas knowing the climate tells you what clothing you need to own.
Life is possible on Earth because the climate is favorable for it to flourish. In particular, the average range of surface temperatures on Earth allows for abundant liquid water, which is essential for life as we know it. Earth’s average surface temperature has changed over time; for example, average global temperature may have been as much as 11°C (20°F) higher than today during parts of the Mesozoic Era, the time of the dinosaurs. But for most of Earth history it has fluctuated within a range that allows for liquid water in the oceans and in land water bodies. Temperature on our planet is controlled, in part, by the fact that we have a thin atmosphere of gases surrounding us; this thin layer acts to hold in some of the energy that the Earth radiates after being warmed by sunlight.
Our two closest neighbor planets, Mars and Venus, have very different atmospheres from Earth, and hence, very different surface temperatures. Venus is similar to the Earth but is closer to the sun, and the additional heat has caused what we call a “runaway greenhouse effect” in which increased temperatures increased the levels of greenhouse gases (learn more here), which further increased the temperature, which further increased greenhouse gases, and so on. The end result is that the temperatures on Venus now can exceed 450° C (842° F) and the atmosphere is nearly 100 times denser than the Earth’s atmosphere. The atmosphere surrounding Venus is 96% carbon dioxide (CO2). Mars, farther than Earth from the sun, has an atmosphere similar to Venus, with 95% CO2, but the total atmosphere is much less dense than that on Earth, about 50 times less dense than the air at the top of Mount Everest. With such a thin “blanket,” little total heat is trapped, thus the average surface temperature on Mars is -53°C (-63°F). The relationship between Venus, Earth, and Mars is an example of what has been called the Goldilocks Principle—the temperature on Earth is not too hot and not too cold, but “just right” for life to exist.
While greenhouse gases keep the Earth’s surface warmer than it would be otherwise, other factors also affect the Earth’s surface temperature. Since the equator gets more direct solar radiation than either of the poles, and thus more energy per square meter, the temperature in the tropics is warmer than in the polar regions. Warm air at the equator rises and flows toward the poles, then cools, sinks, and flows back toward the equator. This process is called convection and a zone where the convection process occurs is called a convection cell. The Earth’s rotation forces the poleward-moving air sideways (a phenomenon called the Coriolis effect), so the poleward moving air doesn’t make it as far as the pole, but rather descends in a high pressure band around 30 degrees latitude in each of the northern and southern hemispheres. For related reasons, two additional convection cells, at mid latitudes and high latitudes, form in each hemisphere. These latitudinal convection cells are host, from equator to pole, of the trade winds, westerlies, and polar easterlies. Overall, the global movement of air distributes heat from the equator to the poles and keeps the surface temperature within the bounds currently experienced on Earth.
How Systems Work
A system is a set of parts that interact with each other. Earth’s climate system is made up of all of the objects and processes that have a global impact on climate. To begin to understand a system as complicated as the Earth’s climate system, scientists observe and analyze the components and their interactions and how a change in one component (a forcing factor) impacts the other components (a response).
The term forcing factor refers to factors that cause change; responses are the changes that result. Forcing factors can produce responses at various rates. These rates can be directly proportional to the magnitude of the forcing factor, in which case they are called linear. For example, pushing a merry-go-round harder will result in a linear increase in the speed of the merry-go-round. Or they can be produced in some more complex, non-linear pattern. For example, a small push to a ball at the top of a hill can cause a much larger change if the ball rolls down the hill. A forcing factor might induce a response immediately after it is applied, or only after some period of time has passed (lag). For instance, when grass seed is planted, there is a lag before your yard is covered by grass, and that amount of time is determined by rainfall, exposure to the sun, and other factors. Forcing factors can be applied at a variety of magnitudes (strong or weak) and durations (long or short).
A very important behavior of all systems is feedback between components. Feedbacks can either amplify a behavior (positive or reinforcing feedback) or suppress it (negative or balancing feedback). (Dr. Kim Kastens, in her 2010 article “Going Negative on Negative Feedback,” discusses the confusion often generated by the colloquial meaning of the words “positive” and “negative” and the use of these words in describing feedback in systems. She suggests alternative terms of “reinforcing” and “balancing” feedback. Her article can be found here.)
An example of a positive or reinforcing feedback cycle in the climate system is melting of sea ice. Sea ice forms when seawater freezes at the surface of the ocean, and it is much brighter (more reflective) than the water around it. Since it is bright it reflects much of the incoming sunlight back to the atmosphere. If a little bit of sea ice melts, more ocean water is available to soak up the heat from the sun, and this warmer water melts more of the nearby ice. This process can amplify until the ice is completely melted. At this point the system of sea ice and seawater has crossed a threshold, that is, it has changed dramatically.
An example of negative or balancing feedback is the impact of clouds on global warming. Warm air contains more water vapor than cold air and more water vapor leads to more clouds, so a warmer Earth will have more clouds. However, clouds are white and reflect sunlight, so more clouds will result in less sunlight reaching the Earth, cooling the climate. Clouds have the potential to counteract global warming, although we do not know exactly by how much.
The Earth’s climate is a complex system, meaning that it has many parts with many interactions. Complexity reduces (or at least makes more difficult) the predictability of the overall behavior of the system. Yet, scientists have enough understanding of the physical and chemical laws that govern Earth’s climate system that they can build computer models which can accurately replicate historical climate data. These same models can be run to predict future climates under different scenarios.
Earth's Climate System
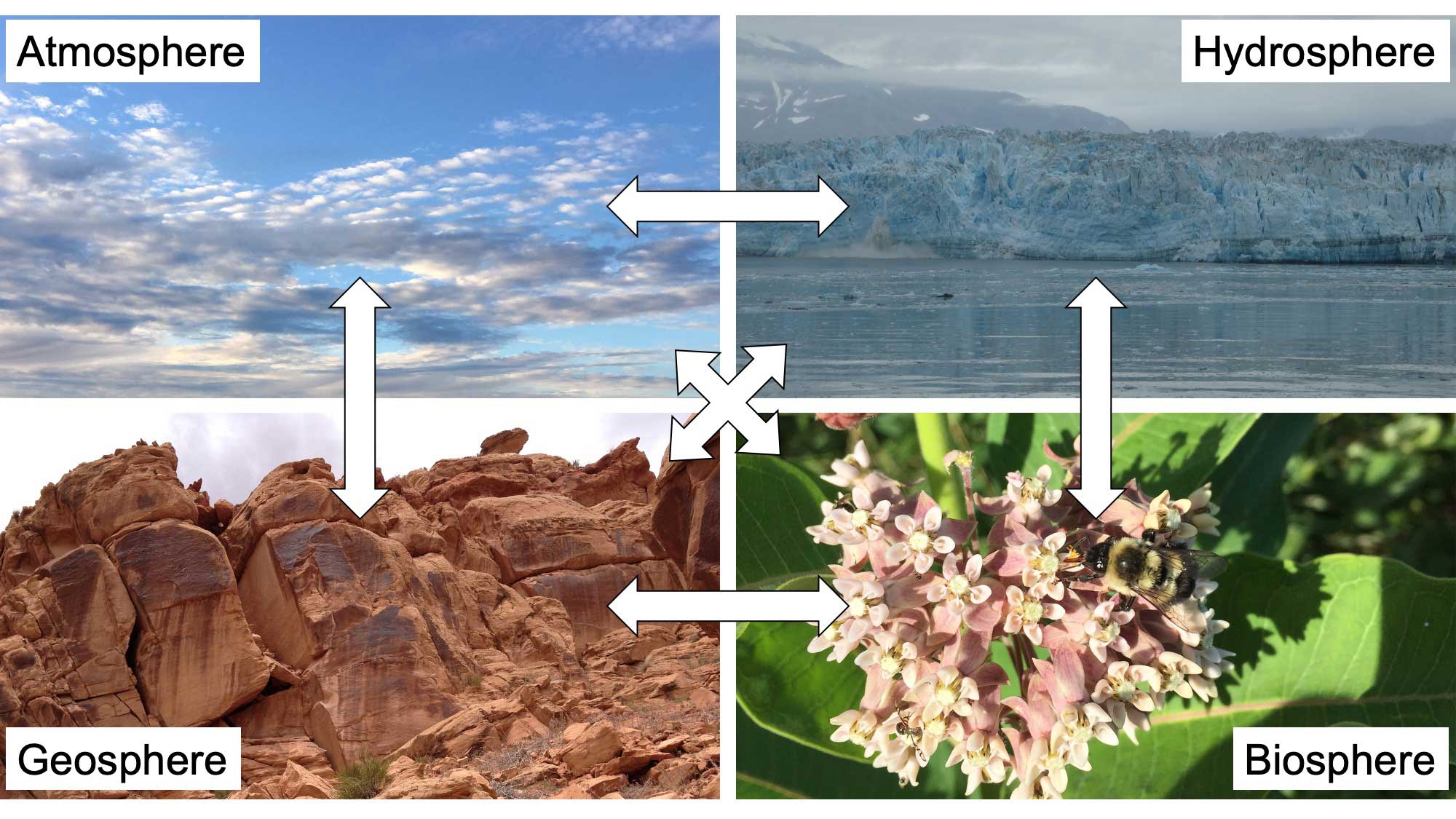
The four connected components of Earth's climate system: the atmosphere, hydrosphere, geosphere, and biosphere. Image created by Jonathan R. Hendricks for PRI's Earth@Home project (CC BY-NC-SA 4.0 license).
The Earth’s climate system consists of air, water, land, and life (or, as they are often called, the atmosphere, hydrosphere, geosphere, and biosphere). Phenomena outside of the Earth (mainly the sun, but also cosmic dust and meteorite impacts) also affect its climate. All of these components interact over time to create the climate conditions that we observe. Life on Earth evolves partly in response to changes in climate, but living systems also influence climate through absorption or emission of greenhouse gases such as CO2 and methane (CH4).
Atmosphere
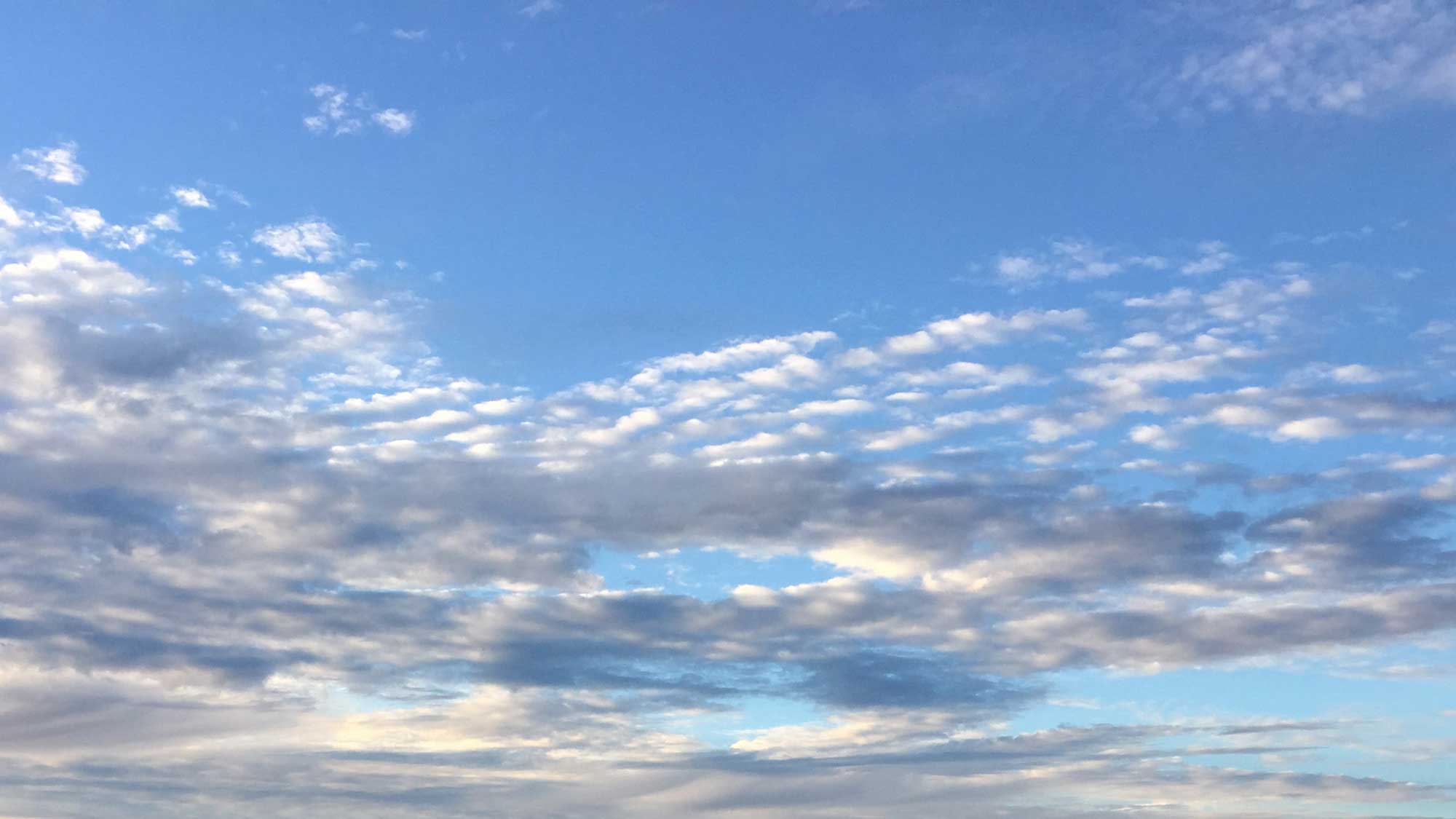
The atmosphere above Cayuga Lake, New York on a summer evening. Photograph by Jonathan R. Hendricks for PRI's Earth@Home project (CC BY-NC-SA 4.0 license).
The atmosphere—the blanket of gas surrounding the Earth (commonly called “air”)—is where most of what we think of as weather and climate happen. Other planets, such as Mars and Venus, also have atmospheres, but they are very different from that on Earth. Our atmosphere consists mostly (approximately 80%) of nitrogen, with oxygen making up most of the rest. Other gases exist in much smaller quantities (see table below) and are called trace gases. Despite their small quantities in terms of percentage of the atmosphere, some of these other gases—such as water vapor, carbon dioxide, methane, and ozone—control the Earth’s climate system because of their influence on temperature: they are greenhouse gases.
Table: Chemical composition of Earth's atmosphere.
Gas | % in Atmosphere |
---|---|
Nitrogen (N2) | 78 |
Oxygen (O2) | 21 |
Water vapor (H2O) | 1 to 4* |
Argon (Ar) | 0.93 |
Carbon dioxide (CO2) | 0.041** |
Neon (Ne) | 0.0018 |
Helium (He) | 0.00052 |
Methane (CH4) | 0.00017 |
Krypton (Kr) | 0.00011 |
Hydrogen (H2) | 0.000055 |
Nitrous oxide (N2O) | 0.00003 |
Carbon monoxide (CO) | 0.00001 |
Xenon (Xe) | 0.000009 |
Ozone (O3) | 0.000007 |
Nitrogen dioxide (NO2) | 0.000002 |
* The concentration of water vapor in the atmosphere varies from about 1% to 4%, depending on the temperature. If temperatures warm, more water vapor can be held in the air, increasing the greenhouse effect from water.
** The concentration of CO2 is rising (414 parts per million as of March 2020); see the most recent data here.
"Face the Trace Gas" by Paleontological Research Institution (YouTube).
Greenhouse gases are not the only components of the atmosphere that affect global temperature. Clouds influence climate in a variety of ways and on a variety of spatial and temporal scales. Clouds can cool the Earth by reflecting sunlight back into space. They can also warm the Earth by absorbing infrared radiation from the Earth and then re-radiating it back downward. The amount of water vapor (and thus of clouds) in the atmosphere is sensitive to temperature: the warmer it is, the more water evaporates, and the more water that the air can hold—approximately 6% more water vapor for every °C of additional heat. This creates a reinforcing feedback in the climate system: the warmer it gets, the more water vapor there will be in the atmosphere, and this will cause still more warming.
Other important compounds in our atmosphere that influence the Earth’s climate are aerosols, which are solid, liquid, or mixtures of solid and liquid particles suspended in the air—from volcanic eruptions, storms, or anthropogenic emissions. Aerosols can cool the Earth by both reflecting incoming sunlight and also serving as “seeds,” or condensation nuclei, for clouds. The number and size of aerosol particles determines whether the water in clouds condenses into a few large droplets or many small ones, and this strongly affects the amount of sunlight that clouds reflect and the amount of radiation that they absorb. The increased reflection of sunlight into space by aerosols usually outweighs their greenhouse effect, but because aerosols remain in the atmosphere for only a few weeks the impact of greenhouse gases in the long run is much more significant.
Hydrosphere
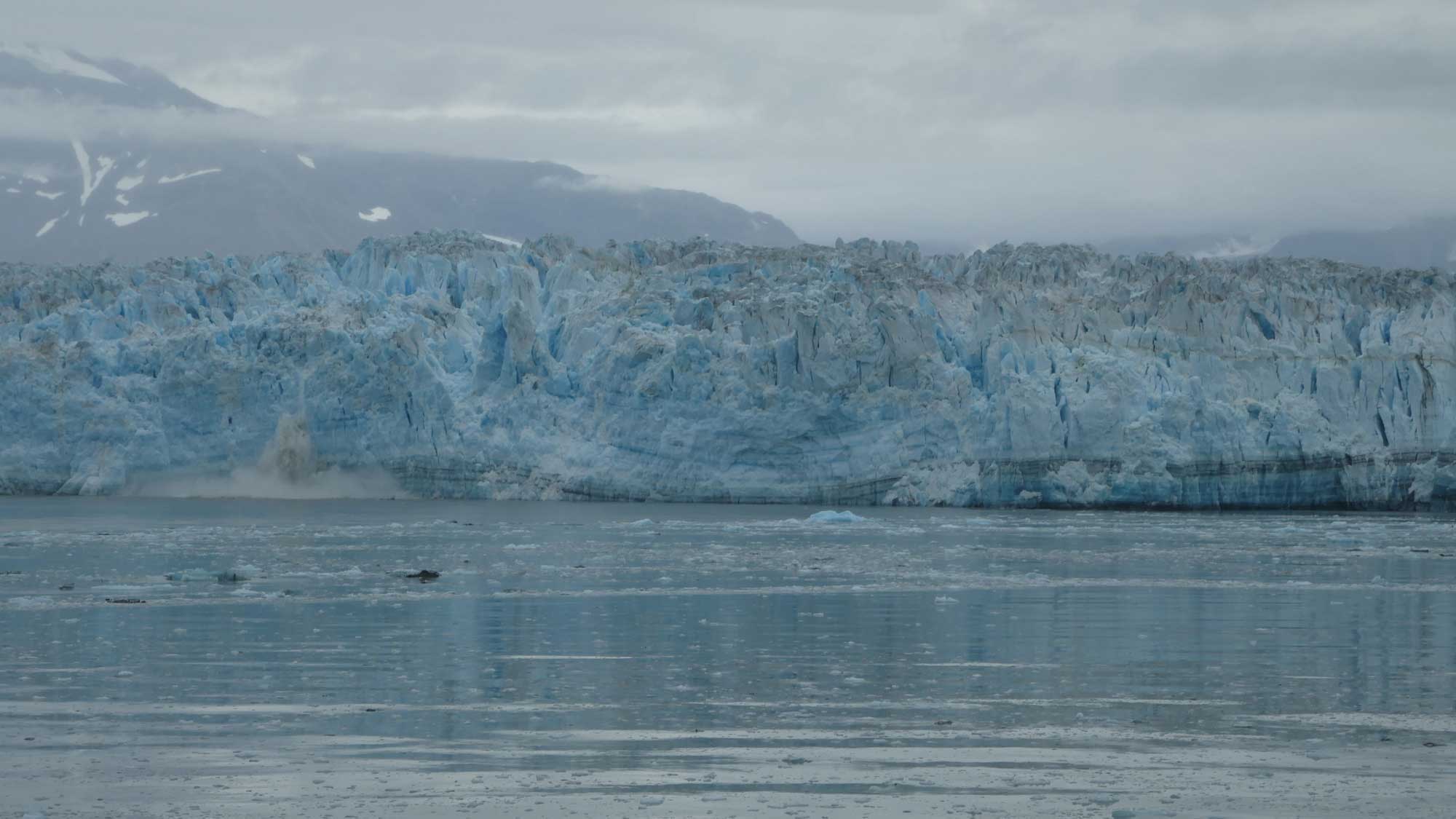
Two components of the hydrosphere visible at Disenchantment Bay, Alaska: liquid water (ocean) and ice (Hubbard Glacier). Photograph by Jonathan R. Hendricks for PRI's Earth@Home project (CC BY-NC-SA 4.0 license).
The hydrosphere includes all the liquid and frozen water at the Earth’s surface. The oceans contain approximately 97% of the water on Earth. Because water holds heat for longer than land, the oceans play a very important role in storing and circulating heat around the globe. The currents in the oceans, in fact, are driven primarily by differences in density, influenced by temperature and also by the salt content. The surface of the ocean receives heat from the sun. This warm water is less dense than colder water found deeper in the oceans. Atmospheric winds and forces that result from the rotation of the Earth create ocean currents that allow cold bottom water to well up to the surface. In the tropics, most of the warm water at the surface is pushed by wind to the centers of large rotating masses of water called gyres.
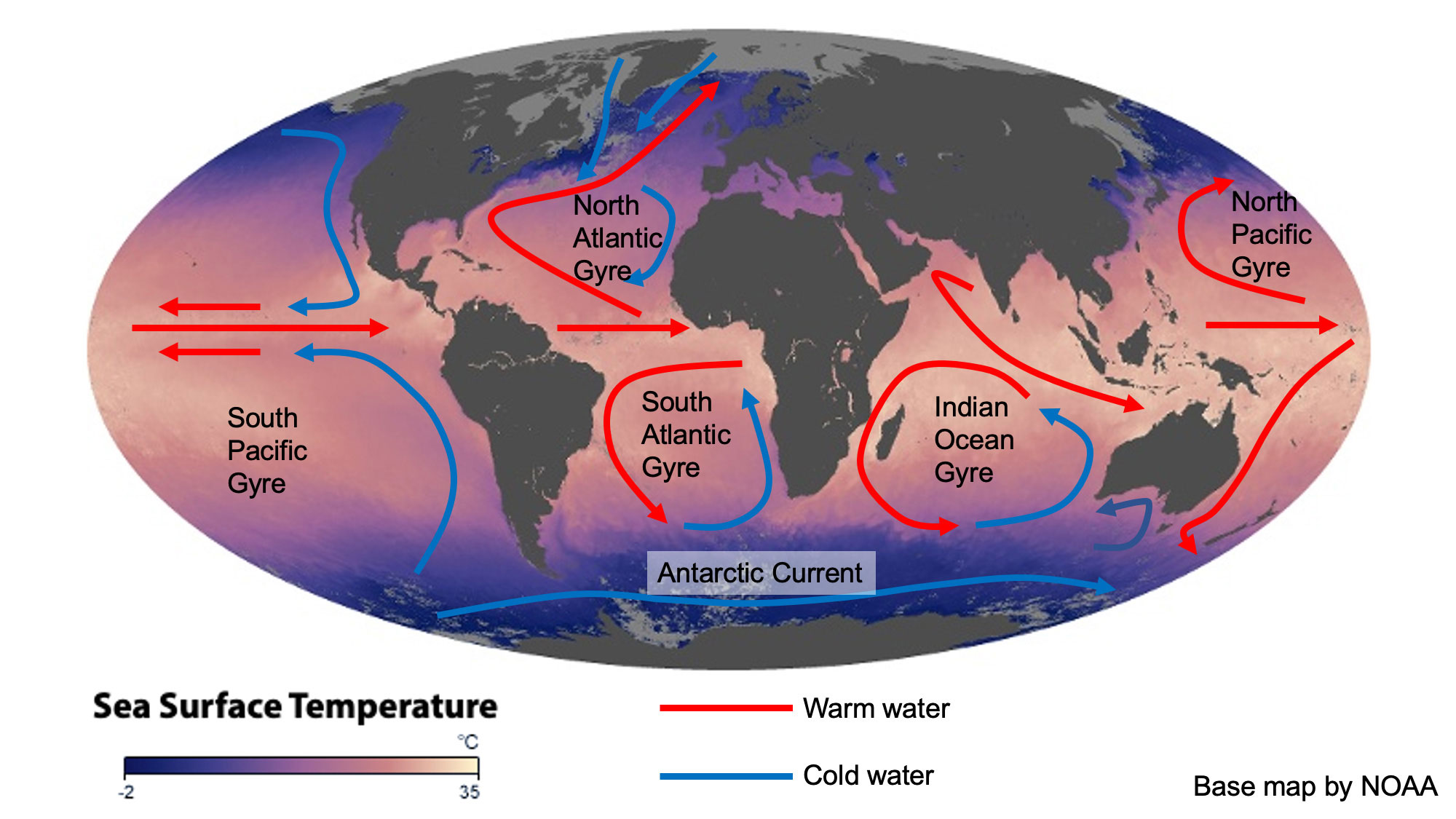
Modern ocean surface circulation. Ocean currents play a huge role in transporting heat energy from equatorial regions to temperate and polar regions. Surface circulation of a relatively thin layer of water is driven by the wind and by the Coriolis force, an effect of rotation of the Earth, which drives gyres in the Atlantic and Pacific Ocean. Subsurface circulation, which is not shown, is driven by cold salty water that sinks near the poles, especially in the North Atlantic. When the warm water begins to cool at higher latitudes, such as around northern Europe, its heat is lost to the atmosphere, contributing significantly to warming the air. Land near water in these regions is therefore usually warmer than land far from the coast. For instance, the average yearly temperature in London is 14°C (57°F). At the same latitude across the Atlantic in Calgary, Alberta, Canada, the average yearly temperature is 4°C (39°F). This is because the Gulf Stream carries warm water from near the equator in the Atlantic Ocean northeast to London, but Calgary is in the middle of North America, far away from the moderating influence of ocean currents. Image created by Jonathan R. Hendricks for the Earth@Home project (CC BY-NC-SA 4.0 license); base map by NOAA (public domain).
Some of the water, such as the Gulf Stream, moves toward the poles. When warm water approaches the poles, it mixes with colder water. Evaporation and formation of sea ice leave behind slightly saltier surface water. This cold, salty sea water, which forms especially in the North Atlantic and near Antarctica, is relatively dense and sinks. It then begins to move under the surface back toward the equator, sliding underneath the warmer and less dense surface waters. This is the primary driver of deep ocean circulation, which can take hundreds or thousands of years to complete one cycle.
The movement of ocean currents, carrying heat energy to different parts of the globe and transferring energy to the atmosphere, plays an extremely large role in global climate. Therefore, the configuration of the continents, around which the ocean currents flow, also plays a large role in their respective regional climates.
Ice at the Earth’s surface includes sea ice, glacier, and continental ice sheets, which altogether hold approximately 2% of the water on Earth. Scientists refer to this system as the cryosphere. Sea ice forms when seawater freezes at -1.9°C (29°F), which is lower than “freezing” (0°C or 32°F) because of the salt content. Like all ice, frozen seawater is less dense than liquid water, and floats atop it. Sea ice acts as an insulating barrier that prevents the ocean from interacting with the atmosphere. When ice is present, heat from the ocean is not lost to the atmosphere, and the water can remain much warmer than the air. Glacial ice occurs as mountain glaciers or continental ice sheets. Mountain glaciers can occur anywhere in the world, but in the tropics they cannot form below 5 kilometers (about 16,400 feet) altitude, where it is too warm.
There are currently two continental ice sheets on Earth, covering most of Greenland and Antarctica. These large continental glaciers lock up great quantities of water that would otherwise be in the ocean, resulting in lower sea levels. If these ice sheets were to melt entirely, global sea level could rise as much as 70 meters (approximately 230 feet). Ice also affects climate itself through its albedo. Albedo is the reflectivity of a surface; high albedo means that a surface is very reflective of light energy, and low albedo means that it absorbs light energy. Ice has high albedo compared with the ocean or land; it reflects back a high percentage of sunlight into the atmosphere, cooling the surface. Continental glaciers can be thousands of feet thick, and can therefore also actually block or redirect air flow, causing warm air to deflect away from the area covered by the ice sheet, and preventing or slowing the warming process.
Geosphere
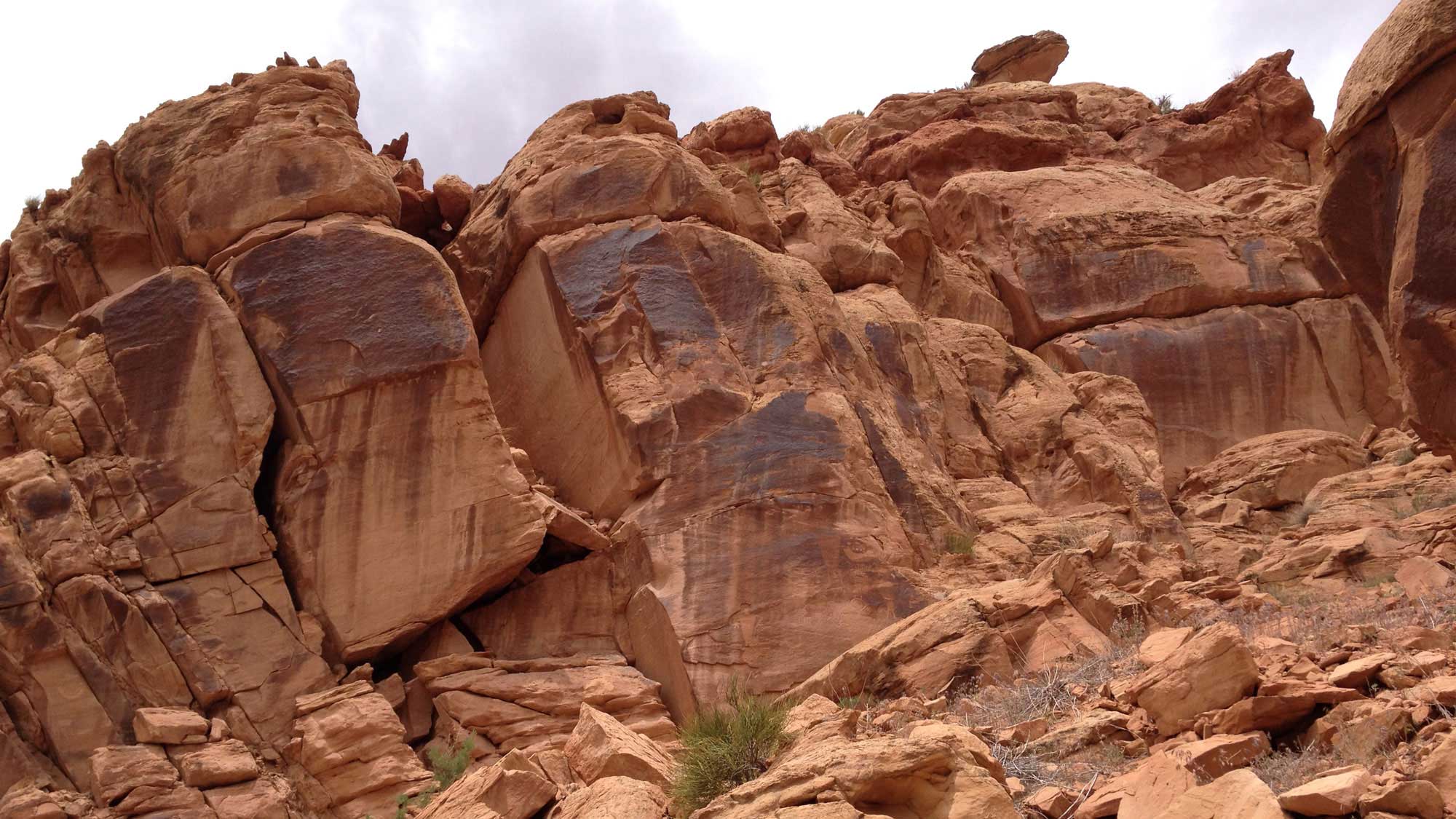
Jurassic-aged rocks exposed at Dinosaur National Monument, Utah represent the geosphere. Photograph by Jonathan R. Hendricks for PRI's Earth@Home project (CC BY-NC-SA 4.0 license).
The geosphere is the solid Earth, from the surface to the core. We might not often think of rocks as being connected to the atmosphere, but they very much are, especially over long stretches of time. For example, even though oxygen makes up 20% of the atmosphere, there is about 200 times more oxygen in the crustal rocks below the surface of the Earth. Exposed rock reacts with atmospheric oxygen by absorbing it, removing oxygen from the atmosphere. The solid Earth affects the climate in many ways. Volcanic eruptions can put large amounts of gas and particles into the atmosphere. Particulates temporarily suspended in the atmosphere can affect how much of the sun’s heat reaches the surface and how much of that is retained; after a large volcanic eruption, the global climate may cool by a few degrees for several years. The different land surfaces have different albedos, variously absorbing and reflecting energy from the sun. Further, sediments and rocks hold a large amount of the Earth’s carbon, impacting the concentration of CO2 in the atmosphere.
Biosphere
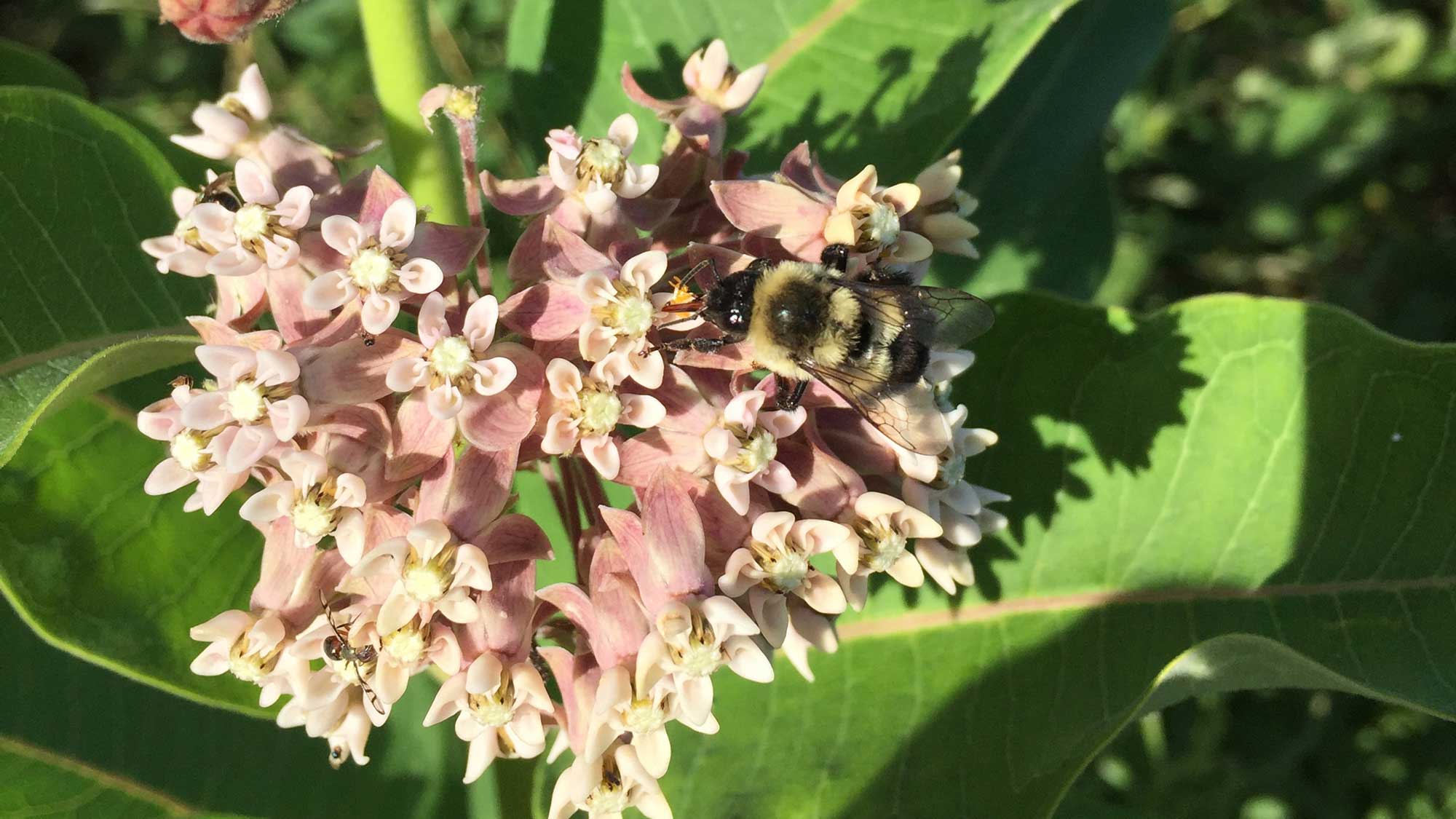
All lifeforms—from bacteria to plants and animals—are part of the biosphere. Photograph by Jonathan R. Hendricks for PRI's Earth@Home project (CC BY-NC-SA 4.0 license).
The biosphere includes all of the life on Earth. Life on Earth is more than just a green layer sitting passively on the surface of a rocky ball: life is an integral part of the geology and climate of the planet. Living things have enormous effects on many atmospheric, oceanic, and geological processes. For example, soil is a byproduct of life; without organic matter, soil would be no more than rock dust (like on the moon). Life also profoundly affects the atmosphere. It is only because of the photosynthetic activity of green plants, along with small organisms like protists and bacteria, that the Earth’s atmosphere contains so much oxygen. These organisms can also act as sinks for the carbon that they contain when they die and are buried in sediment that may become rock.
A wide range of organisms help to cycle carbon back to the atmosphere. Plants stabilize the land and limit physical weathering (erosion) from wind and water and simultaneously contribute to chemical weathering of rocks by changing the acidity of the soil. Animals (not to mention humans) alter the landscape in a wide variety of ways, from churning up seafloors and soils to building major structures like coral reefs, beaver ponds, and termite mounds. The remains of dead plants, animals, and microbes form vast deposits of sediment that become layers of rock in the Earth’s crust. All of the coal, oil, and natural gas and most of the limestone in the world, for example, were formed by the accumulated body parts of once-living things.
Regional Weather and Climate Systems
Just as weather and climate are affected globally by the placement of continents and oceans, smaller features such as topography and local human land use can affect regional weather and climate patterns, frequently making it difficult to predict what effect climate change will have in a given area. It is also difficult to extrapolate from such regional patterns to global patterns. These regional features create regional and local effects, such as heat island, rain shadows, and lake effect snow.
Heat islands occur in urban areas, with the result that such areas are often warmer than nearby rural areas. The building materials used to create metropolitan structures absorb heat in the day and then release it at night. This can cause some urban areas in extreme circumstances to be up to 9°C (16°F) warmer than their rural counterparts.
Rain shadows refer to the areas adjacent to a mountain range that receive little rain. The mountains separate the area in a rain shadow from a significant water source, like an ocean. As warm air moves over the ocean it collects water in the form of water vapor, which can run into a mountain range where it is forced upward. When air is forced upwards, it expands and cools. Warm air can contain more water vapor than cool air, so as this air cools water vapor condenses out to form clouds and rain. The rain falls on the windward side of the mountains closer to the water source, leaving the opposite side (leeward) of the mountains and adjacent areas very dry, in a rain shadow.
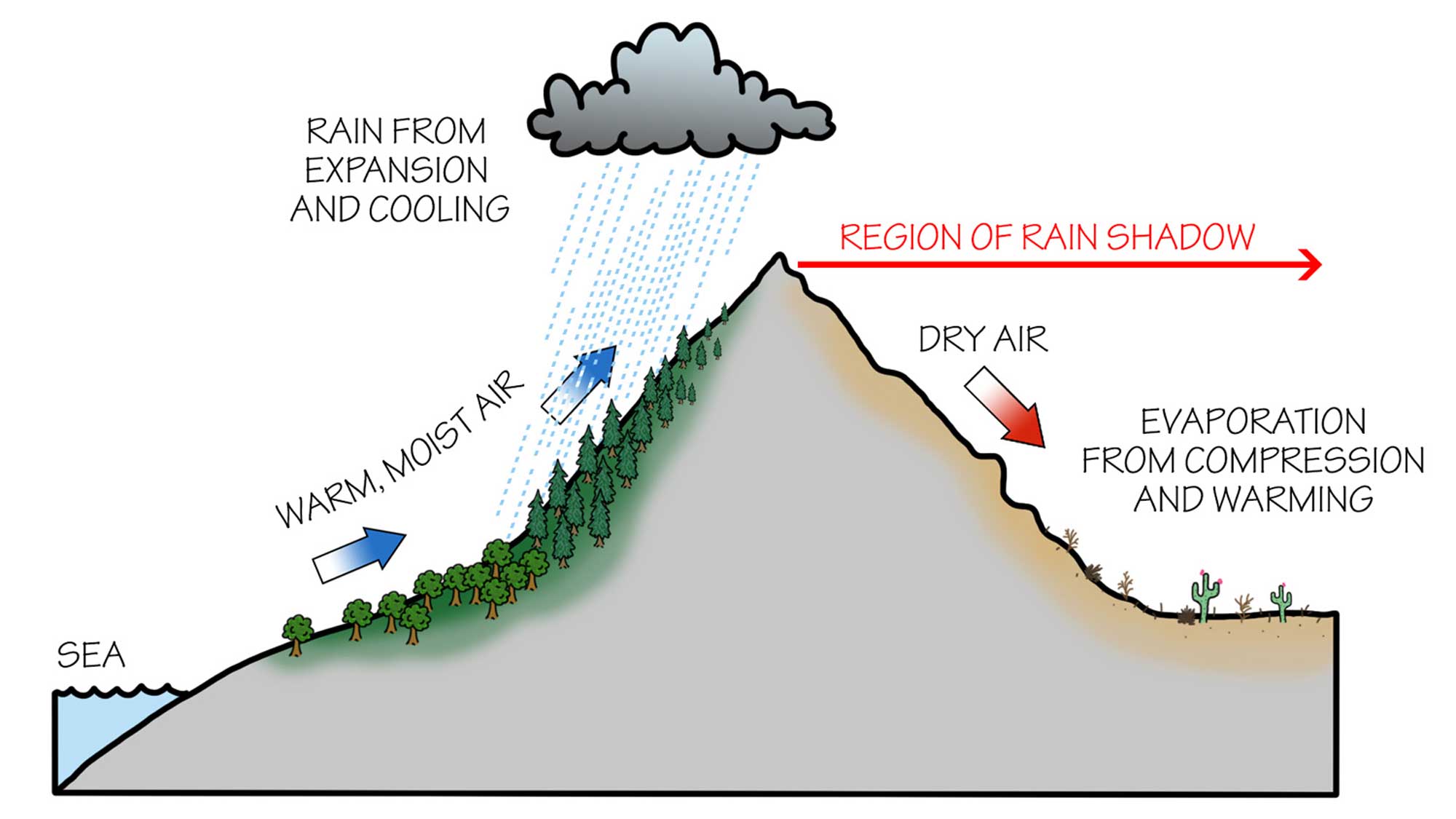
Key characteristics of a rain shadow. Image by Wade Greenberg-Brand for the Paleontological Research Institution (CC BY-NC-SA 4.0 license).
Lake effect snow refers to a type of snowfall pattern in which cold air flows over the warmer water of a large lake. As water evaporates from the lake it interacts with the cold air, forming clouds over the lake. These then get carried to shore by the winds, which deposit snow (sometimes a lot of snow) on land in the path of the winds. Regions along the eastern shores of large lakes, such as the eastern shores of Lake Michigan (Traverse City and Grand Rapids), Lake Erie (south of Buffalo) and Lake Ontario (Syracuse and Watertown), can have significantly different snowfall patterns than areas farther from the lakes.